The Quantum Diamond Microchip Imager (QDMI) is an advanced imaging technology that leverages quantum properties of diamond to achieve highly sensitive magnetic and electric field detection. Here’s a detailed explanation of the QDMI and its practical applications:
Atomic Structure of NV Centers in Diamond
- Diamond Lattice:
- Diamond is a crystalline form of carbon where each carbon atom is tetrahedrally bonded to four other carbon atoms, creating a strong and rigid lattice structure.
- NV Center Defect:
- Nitrogen Atom: A nitrogen atom replaces one of the carbon atoms in the diamond lattice.
- Vacancy: Adjacent to the nitrogen atom is a vacancy, which is a missing carbon atom in the lattice.
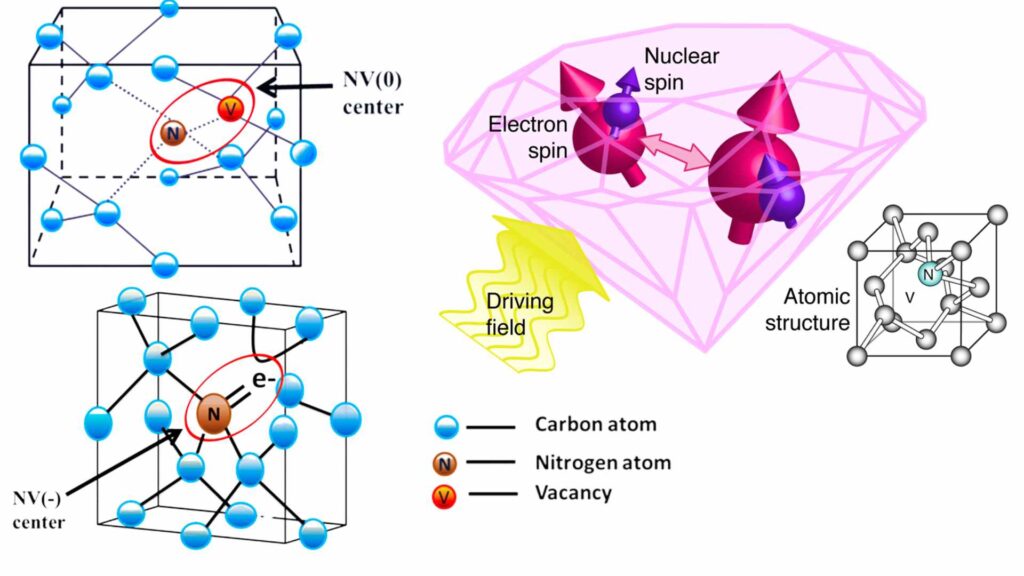
Quantum Properties
NV centers have unique quantum properties that allow them to be used as quantum sensors. They exhibit spin states that can be manipulated and read out using optical and microwave techniques.
- Spin States:
- The NV center exhibits electron spin states that can be manipulated using magnetic and electric fields. The most commonly used spin states are the 𝑚𝑠=0ms=0 and 𝑚𝑠=±1ms=±1 states.
- These spin states can be optically pumped, meaning they can be excited and read out using specific wavelengths of light (typically green laser light for excitation and red for fluorescence readout).
- Optical and Microwave Techniques:
- Optical techniques involve using laser light to excite the NV center and read out its spin state based on the emitted fluorescence.
- Microwave techniques are used to manipulate the spin states. By applying microwave radiation at specific frequencies, the spin states can be coherently controlled.
Quantum Diamond Microchip Imager (QDMI)
Principle and Technology:
1. Diamond NV Centers
- Nitrogen-Vacancy (NV) Centers: The core technology of QDMI involves nitrogen-vacancy centers in diamonds. NV centers are defects in the diamond lattice where a nitrogen atom replaces a carbon atom next to a vacancy (a missing carbon atom).
- Quantum Properties: These NV centers have quantum properties that are sensitive to magnetic fields, electric fields, temperature, and strain, making them excellent for various sensing applications.
2. Quantum Sensing
- Magnetic Field Detection: QDMI can detect very weak magnetic fields with high spatial resolution due to the quantum properties of NV centers. This makes it useful in fields such as neuroscience for mapping neural activity and in material science for studying magnetic materials.
- Temperature Sensing: The NV centers can also act as highly sensitive thermometers, allowing for precise temperature measurements at the nanoscale.
3. High Resolution and Sensitivity
- Nanoscale Imaging: The spatial resolution of QDMI can reach the nanometer scale, providing detailed images of structures and phenomena that are otherwise difficult to observe with conventional imaging technologies.
- Sensitivity: The high sensitivity of NV centers to magnetic and electric fields enables the detection of very small changes in these fields, allowing for detailed imaging of subtle phenomena.
4.Magnetometry:
- NV centers are sensitive to magnetic fields. When exposed to a magnetic field, the energy levels of their electron spins split in a way that can be measured precisely.
- By optically pumping the NV centers and using laser light to read their spin states, the QDMI can create high-resolution magnetic field maps.
5.Electric Field Sensing:
- Similarly, NV centers can be used to detect electric fields by monitoring shifts in their spin states caused by electric field interactions.
6.Temperature Sensing:
- NV centers are also sensitive to temperature changes, allowing for temperature mapping at the nanoscale.
Applications
- Biological Imaging: In biology and medicine, QDMI can be used to image cells and tissues with high resolution, potentially leading to advancements in understanding cellular processes and disease mechanisms.
- Material Science: Researchers can use QDMI to study the properties of new materials at the atomic level, aiding in the development of new materials with desirable properties.
- Quantum Computing: The technology also has implications for the development and improvement of quantum computers, as NV centers in diamonds can be used for qubits in quantum computing.
Technology Integration
- Microchip Design: Integrating the NV centers into microchips allows for the development of compact and efficient imaging devices. These microchips can be incorporated into larger systems for practical applications.
- Ease of Use: QDMI systems are being designed to be user-friendly, making advanced imaging technology accessible to a broader range of researchers and industries.
Practical Applications
- Medical Imaging:
- Magnetic Resonance Imaging (MRI): QDMI can be used to enhance MRI technology by providing highly localized magnetic field measurements. This can improve the resolution of MRI scans, particularly useful in neuroimaging and detecting small-scale biological structures.
- Cancer Detection: QDMI can be employed to detect magnetic nanoparticles targeted to cancer cells, offering a highly sensitive method for early detection of tumors.
- Material Science:
- Characterization of Magnetic Materials: QDMI is used to study magnetic domains and properties in materials, essential for developing advanced magnetic storage devices.
- Superconductors: The imager can map the magnetic flux in superconducting materials, aiding in the development of new superconducting technologies.
- Quantum Computing and Information:
- Qubit Characterization: NV centers themselves can be used as qubits. QDMI can help in characterizing and understanding other qubit systems, essential for quantum computing development.
- Quantum Sensors: Beyond imaging, NV centers are used in various quantum sensing applications, such as detecting minute magnetic fields for scientific research.
- Geoscience:
- Magnetic Field Mapping: QDMI can be used for geological surveys by mapping the Earth’s magnetic field with high precision, which is useful in mineral exploration and understanding geophysical phenomena.
- Microscopy:
- Nanoscale Magnetic Imaging: QDMI provides high-resolution images of magnetic fields at the nanoscale, crucial for studying nanomagnetic structures in advanced materials and devices.
Example Applications
- Neuron Activity Mapping:
- By detecting magnetic fields generated by neural activity, QDMI can potentially map brain activity at a much higher resolution than current technologies.
- Live Cell Imaging:
- QDMI can be used to monitor magnetic nanoparticles within live cells, providing insights into cellular processes and drug delivery mechanisms.
- High-Resolution MRI Contrast:
- Enhancing the contrast in MRI scans by detecting the presence of specially designed contrast agents with magnetic properties.
Advantages of QDMI
- High Sensitivity: The quantum properties of NV centers allow for the detection of extremely weak magnetic and electric fields.
- Non-Invasive: Many applications, particularly in medical imaging, benefit from the non-invasive nature of QDMI.
- Nanoscale Resolution: The ability to achieve nanoscale resolution opens up many new avenues in both biological and material sciences.
Challenges and Future Prospects
- Scalability: Integrating QDMI technology into existing systems on a large scale poses technical challenges.
- Optimization: Ongoing research is focused on improving the sensitivity, stability, and ease of use of QDMI.
- Interdisciplinary Research: Combining advances in quantum physics, materials science, and engineering is essential for further development and application of QDMI.
In summary, the Quantum Diamond Microchip Imager represents a cutting-edge intersection of quantum physics and imaging technology, with a wide range of practical applications in medicine, materials science, quantum computing, and beyond. Its development continues to push the boundaries of what is possible in high-resolution, sensitive imaging.
You may Like